Terminology
The IAU definition is quite broad and does not specify orbital qualifications - a planet is defined primarily by its physical attributes, and secondarily by its orbital characteristics. There are more specific terms which derive from "planet" and define more kinds of planetoids. They are also occasionally referred to as "planemos" - short for
planetary-mass objects.
Planets & Planetinos
A "planet" (or "major planet") is a planetoid which directly orbits a
star (or stars). Natural bodies that orbit a star but are
not gravitationally rounded, such as asteroids and comets, are called "
planetinos" (or "minor planets").
Major & Minor Moons
A "moon" is a planetoid or planetino which orbits another planet or planetino; they are formally called "natural satellites." Planetoid satellites are classified as "major moons," in contrast to planetino "minor moons."
Unbound Planetoids
Planetoids which do not orbit any object, planet or star, are officially classified as "unbound planetoids." However, these objects are more commonly called "rogue planets" or "wandering planets."
Planetary Class System
Beyond the initial definition, planetoids are described by a system of classifications split into four categories: size, temperature, atmosphere, and type, expressed as follows:
[temperature] [atmosphere] [size]-
[type]. The planetary class designations of planetary bodies are one-word labels representing particular defining aspects of themselves, intended to convey to the reader a very general but fairly accurate assessment of what the planet is like in each category. A planet's biosphere is classified according to a
separate system.
Size
All telluric and cryonic planetary bodies can be classified as one of the following size classes based on their mass:
- Micro- (< 0.002 MEarth)
- Mini- (0.002 MEarth to 0.02 MEarth)
- Sub- (0.02 MEarth to 0.2 MEarth)
- No prefix (0.2 MEarth to 2 MEarth)
- Super- (2 MEarth to 10 MEarth)
- Mega- (> 10 MEarth)
All nephelic planetary bodies can be classified as one of the following size classes based on their mass:
- Mini- (< 5 MEarth)
- Sub- (5 MEarth to 10 MEarth)
- No prefix (10 MEarth to 25 MEarth)
- Super- (25 MEarth to 50 MEarth)
- Mega- (> 50 MEarth)
All jovian planetary bodies can be classified as one of the following size classes based on their mass:
- Mini- (< 50 MEarth)
- Sub- (50 MEarth to 200 MEarth)
- No prefix (200 MEarth to 600 MEarth)
- Super- (600 MEarth to 1800 MEarth)
- Mega- (> 1800 MEarth)
Temperature
All planetary bodies can be classified as one of the following temperature classes based on their average surface temperature:
- Frigid (< 90 K) - The methane frost-line effective temperature threshold is estimated at 90 K
- Cold (90 K to 170 K) - The water frost-line effective temperature threshold is estimated at 170 K
- Cool (170 K to 250 K) - The lowest effective temperature for a habitable planet is estimated at 250 K
- Temperate (250 K to 330 K) - The highest effective temperature for a habitable planet is estimated at 330 K
- Warm (330 K to 500 K)
- Hot (500 K to 1000 K)
- Torrid (> 1000 K)
Atmosphere
All planetary bodies can be classified as one of the following atmosphere classes based on their surface pressure:
- Airless (< 1e-6 bar)
- Infrabaric (1e-6 bar to 1e-2 bar)
- Hypobaric (1e-2 bar to 1e-1 bar)
- Mesobaric (1e-1 bar to 1e1 bar)
- Hyperbaric (1e1 bar to 1e2 bar)
- Ultrabaric (> 1e2 bar)
Type
All planetary bodies can be classified as one of the following twelve types based on their overall geophysical structure, composition, solvent presence, and atmosphere. Solid planets are generally divided into two phyla depending on their bulk composition:
tellurae are worlds that are >50% silicate mineral or metallic;
cryonae are worlds that are >50% volatiles
[1]. These two solid planet phyla are further classified by the atmosphere and liquisphere properties they exhibit.
Tellurae are typically stratified into three or more layers: a solid inner core, a molten mantle, and a solid crust. They are composed mostly of stable transition metals and silicates, though this is concentrated in the inner layers (especially the core). The crust of telluric planets, by contrast, consist largely of lighter metalloids and nonmetals (such as silicon, carbon, and aluminum). Telluric planets often have tectonic activity caused by interior magma cycles or, in some cases, extreme heating and cooling of the surface.
Cryonic planets, by contrast, are enveloped in glacial shells rather than rocky crusts, which may span all the way to the planets' smaller, lighter cores, often of porous rock; some cryonae even have undifferentiated interiors of jumbled rock in ice. Occasionally, however, these icy crusts hide global liquid oceans, akin to the molten mantles of tellurae, that produce tectonic activity in the glacial crust. These subsurface oceans are warmed by either a core dynamo effect between the core and whatever thin mantle may exist, or tidal heating in certain cases.
The other two major classes of planet are
nephelae and
joviae. Nephelae are planets with supercritical
[2] atmospheres composed of volatiles, while joviae are characterized by hydrogen-helium-dominant atmospheres. These types of planets, unlike solid worlds, are characterized by a density gradient toward their cores. Typically the stratification of supercritical and gaseous planets includes a solid metallic core at the center, surrounded by a hot, supercritical mantle which is in turn enveloped by an atmosphere of gaseous hydrogen, helium, and volatiles.
Selenae
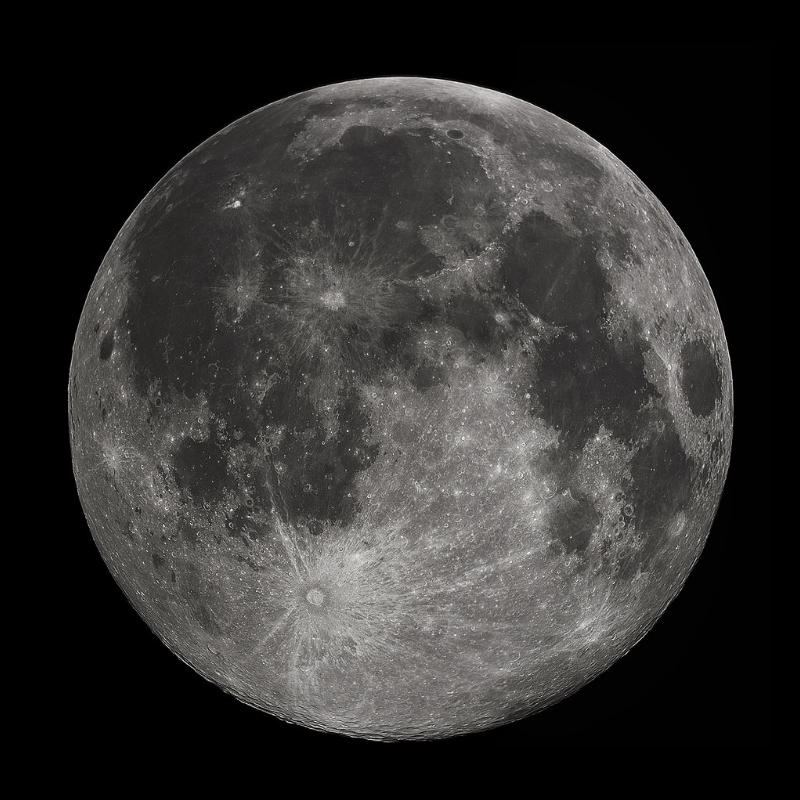
by Gregory Rivera
Luna, the selenic planet archetype.
Selenic planets (designated by
s) are telluric worlds with extremely thin to nonexistent atmospheres. Because of this, their rough surfaces remain unweathered, though they are subject to extreme temperature fluctuations between sunlight and shadow. A selenic planet with endemic life is unheard of, though technically not impossible. Chemosynthetic or photovoltaic autotrophs could form colonies on the airless surfaces of selenae, though this is as yet unproven.
Desertae
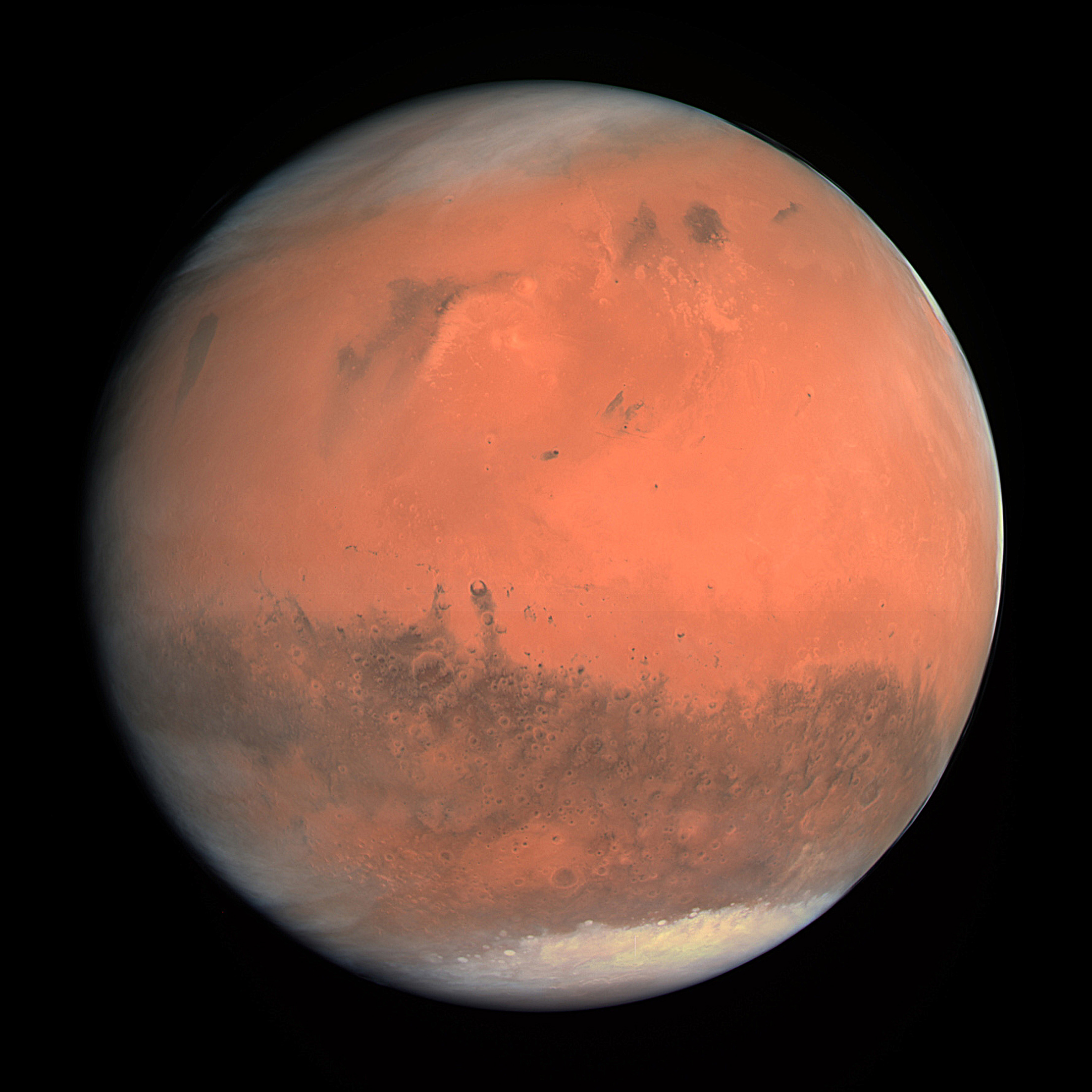
by ESA (Rosetta)
Mars, the desert planet archetype.
Desert planets (designated by
d) are worlds with an overall arid climate and scarce precipitation or surface solvent. This is not to say desertae are entirely devoid of solvent; it often exists under the surface, inside permanent polar ice caps, or even on the surface in liquid state. However, desertae are defined as having less than one third of their surface covered by liquid solvent, often much less. Life is not common on desert worlds, but exists in relative abundance; typically organic but occasionally exotic in nature.
Terrae
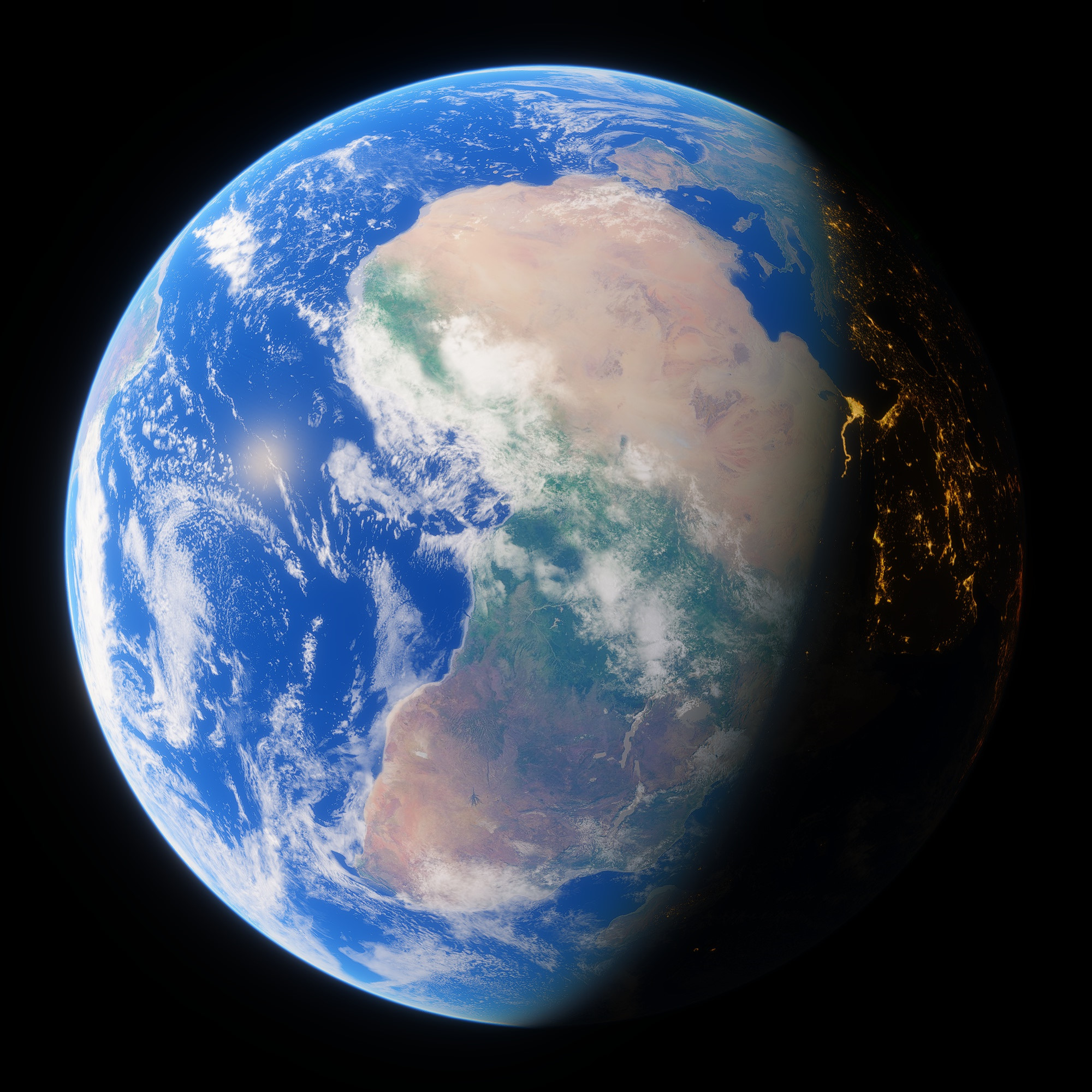
by UNAC (SEO-44) / Doug Marshall
Earth, the terran planet archetype.
Terran planets (designated by
tr) are telluric worlds with a significant amount of liquid solvent present on the surface; specifically, more than one third of the surface. Life is common on terrae, typically organic but with the occasional exotic biosphere. The presence of life maintains the oceans and atmosphere, just as the oceans and atmosphere allow for the presence of life in the first place. In light of this elaborate circular dance of geology and biology, the most complex planetary bodies are usually terrae.
Vulcanae
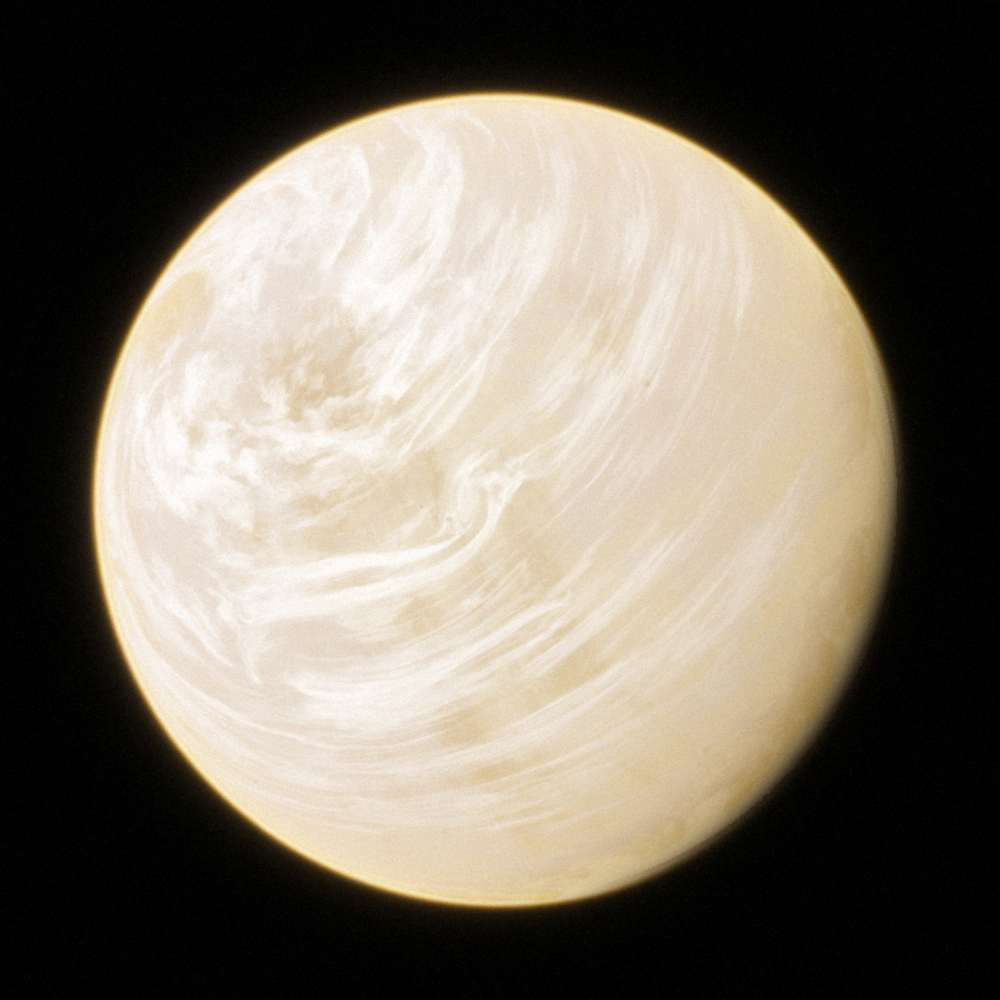
by UNAC (Sojourn 2) / Doug Marshall
Vulcan, the vulcanic planet archetype.
Vulcanic planets (designated by
v) are a subset of telluric worlds with ultrabaric atmospheres, and can range from being completely arid to oceanic (although in that case, they verge toward being nephelae). They are usually extremely hot due to intense greenhouse effects, and on occasion have seas of molten rock. Vulcanic worlds rarely have endemic life, but some have unicellular biospheres composed of exotic extremophiles that thrive in the heat and high pressures.
Erimae
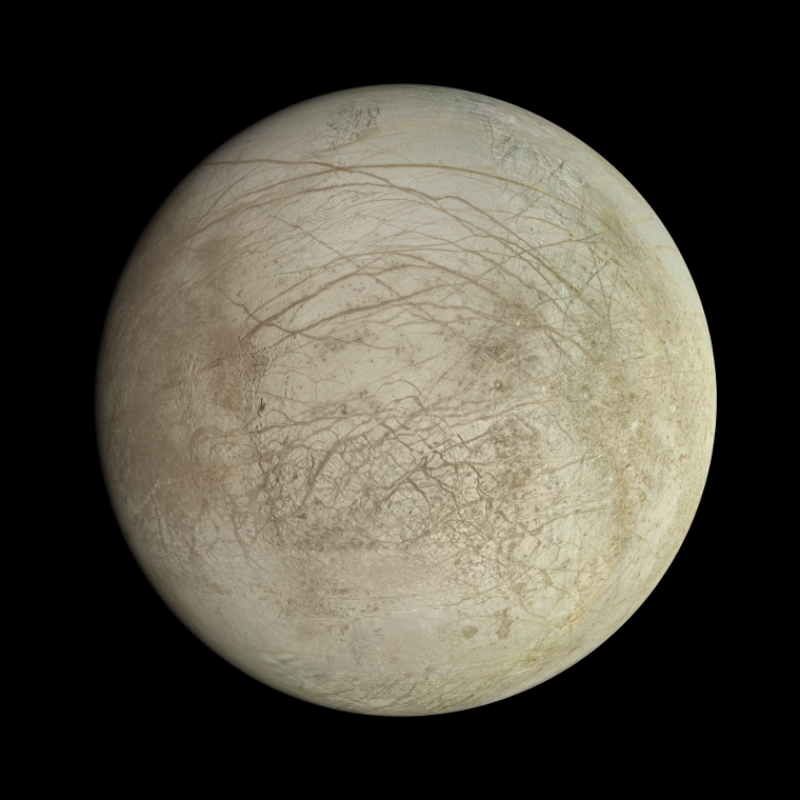
by NASA (JPL) / Bjorn Jonsson
Europa, the erimic planet archetype.
Erimic planets (designated by
e) are airless worlds, similar to
selenae, which are primarily composed of solidified volatiles. Erimae are exposed to the same intense temperature fluctuations as their telluric counterparts, though this does not melt the ices at the surface due to the lack of atmosphere. Erimae sometimes have native life, most often in whatever liquid ocean exists beneath the icy crust but occasionally playing host to surface-dwelling microbes.
Chionae
Titan, the chionic planet archetype.
Chionic planets (designated by
c) are the cryonic counterpart to
desertae: they possess atmospheres ranging from infrabaric to hyperbaric, and less than one third of their surfaces are covered by liquid solvent. Like other cryonic worlds, chionae frequently possess interior oceans as well, and occasionally these subglacial seas breach the surface. Life is not common on erimic worlds, but does exist; typically exotic in nature due to the bizarre chemical and energy environments typical of cryonae.
Aquarae
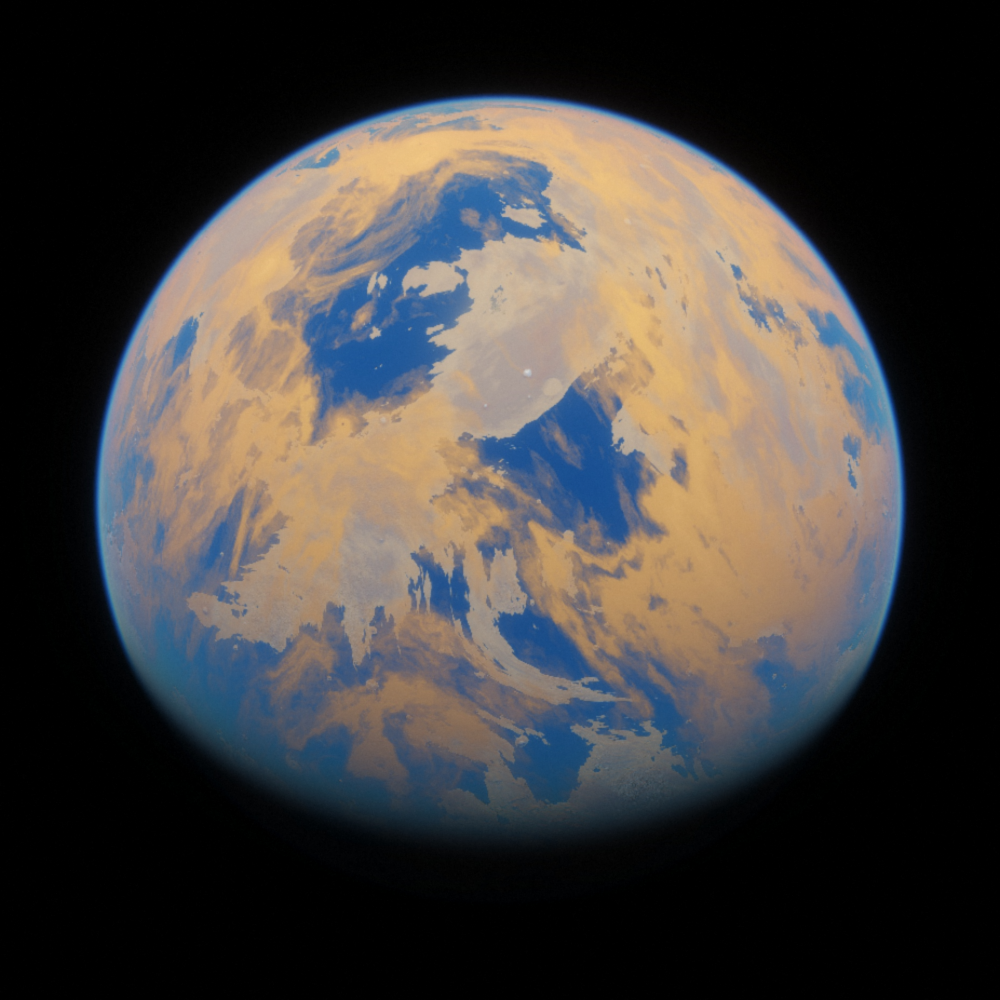
by UNAC (Sojourn 2) / Doug Marshall
Juventas, the aquaric planet archetype.
Aquaric planets (designated by
q) are the cryonic counterpart to
terrae: they possess atmospheres ranging from infrabaric to hyperbaric, and more than one third of their surfaces are covered by liquid solvent. Like other cryonic worlds, aquarae frequently possess interior oceans as well, and these subglacial seas often breach the surface. Life is relatively common on aquaric worlds, but is typically exotic in nature due to the bizarre chemical environments and low energy systems typical of cryonic planets.
Tethae
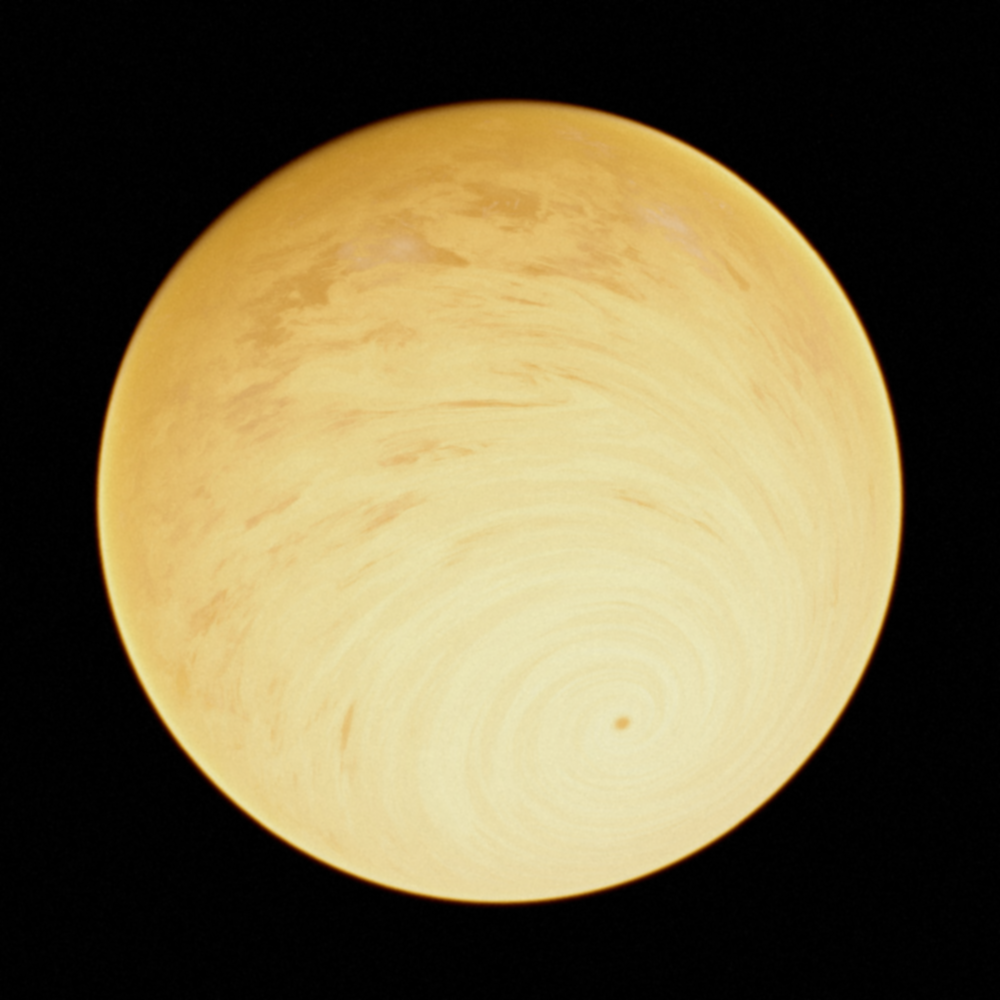
by UNAC (DSASF) / Doug Marshall
Echidna, the tethian planet archetype.
Tethian planets (designated by
th) are a subset of cryonic worlds with ultrabaric atmospheres, and can range from being completely arid to oceanic (although in that case, they verge toward being nephelae). These worlds are often quite hot, though the surface still remains solid due to the extreme ambient pressure. Tethian worlds rarely have endemic life, but some have unicellular biospheres composed of exotic extremophiles that thrive in the high pressures and strange chemistry.
Oceaniae
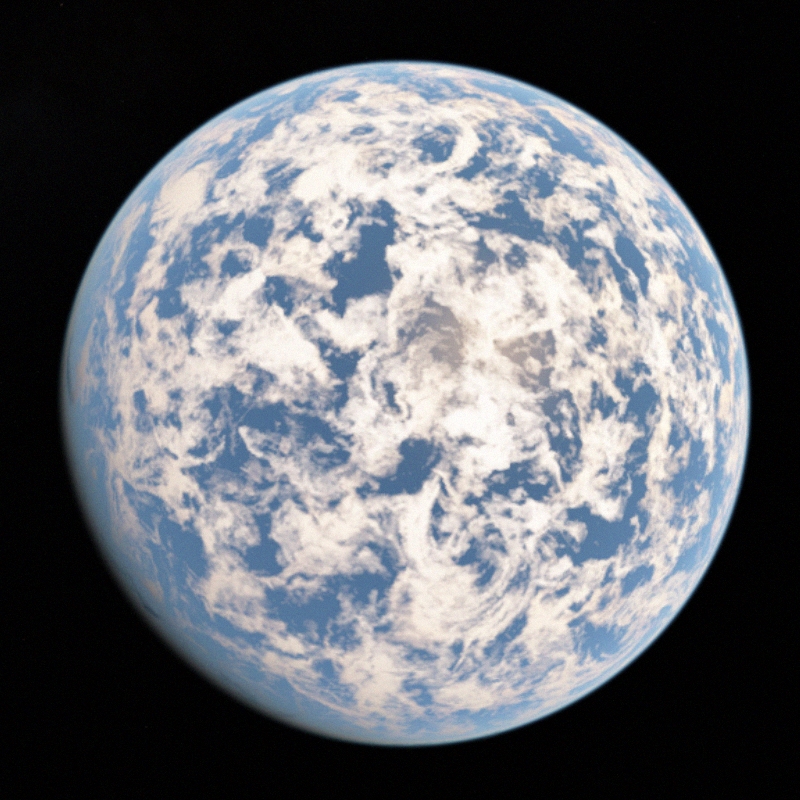
by UNAC / Doug Marshall
Oceanus, the oceanic planet archetype.
Oceanic planets (designated by
o) are worlds whose surfaces are completely covered by solvent, and often a substantial portion of their mass is said solvent. The global seas lay atop a dense nickel-iron and/or silicate core, which is typically surrounded by a mantle of high-pressure ice. The solvent mass of oceaniae is almost always water, though an ammonia oceanic world exists:
Tiamat in the
Altair system. Life on oceanic worlds is common and virtually always organic.
Nephelae
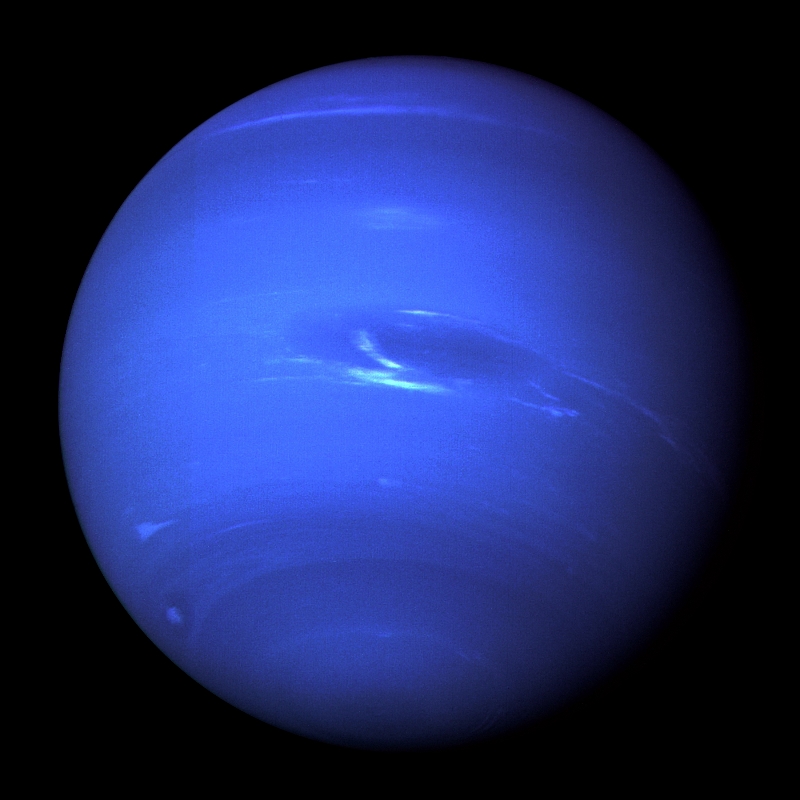
by NASA (JPL - Voyager 2)
Neptune, the nephelic planet archetype.
Nephelic planets (designated by
n), also called ice giants, are vaporous worlds composed primarily of volatiles in a gaseous or often supercritical state. In essence, ice giants occupy the planetary spectrum between oceaniae and joviae. Life on nephelae is quite rare, and tends to be exotic more often than organic: based on nitrogen, phosphorus, and other alternative biochemistries. Most nephelic biospheres are aerial in nature, though in denser strata the inhabitants function more like marine life.
Joviae
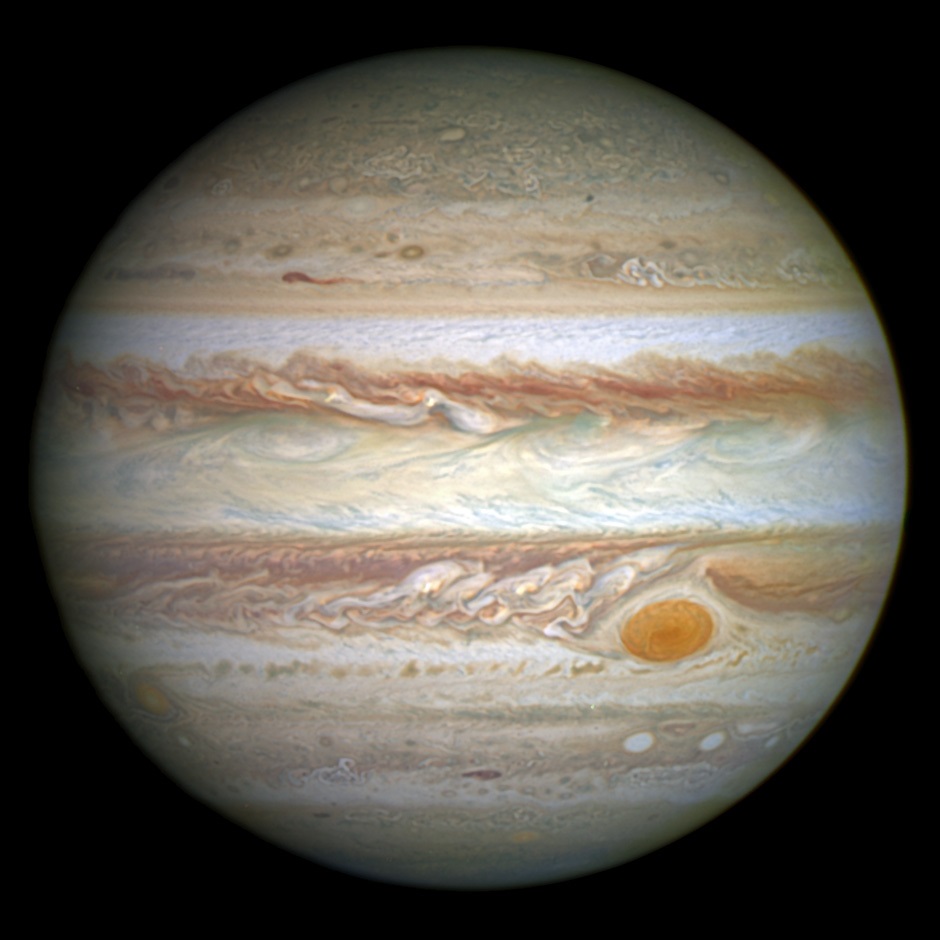
by NASA/ESA (Hubble Space Telescope)
Jupiter, the jovian planet archetype.
Jovian planets (designated by
j), also called gas giants, are worlds composed primarily of gaseous hydrogen and helium. In jovian planets, the mantle is composed of supercritical hydrogen and helium, gradually fading to a vast hydrogen-helium atmosphere. The outermost layers of joviae are the most complex and beautiful: trace compounds form an ever-shifting, multicolored labyrinth of cloud strata and cyclones. Life on joviae is very rare and almost always exotic in nature, though that of
Bellerophon is organic.
Cthoniae
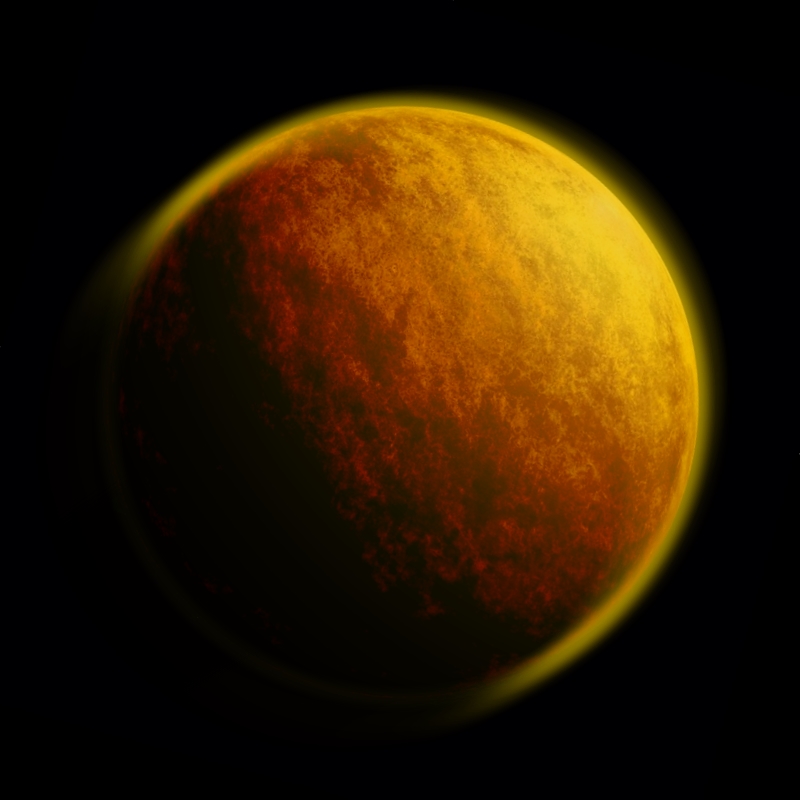
by UNAC (DSASF) / Doug Marshall
Icarus, the cthonic planet archetype.
Cthonic planets (designated by
ct) are a special, rare class of torrid worlds with extremely high metallic and metalloid content. Very few are known, but in the case of
Icarus, the life cycle of cthonic planets is evident: cthoniae are the remaining cores of
joviae whose atmospheres have been stripped away by solar winds, usually because of close proximity to their star. A cthonic world with endemic life is unheard of, though technically not impossible with exotic biochemistry.
[1] "Volatiles" are chemical compounds with freezing points above about 100 K, such as water, methane, ammonia, or the carbon oxides.
[2] Supercritical fluids occur when a substance is subjected to a temperature and pressure high enough that distinct liquid and gas phases do not exist. It can effuse through solids like a gas, and dissolve materials like a liquid.
Related Articles
This article is beautifully structured and amazingly well-written. You've done an awesome job, especially with links! I didn't feel confused at all.
Thank you!! I'm really happy you found it easy to read and understand. :D